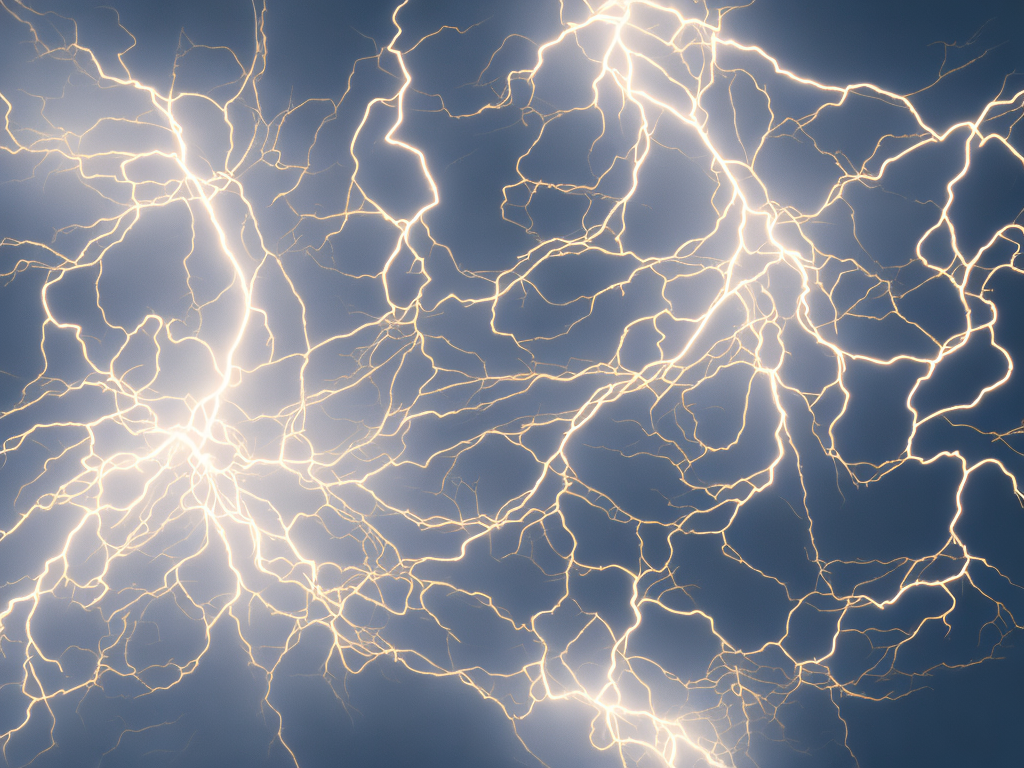
When a charge is in motion, it produces a magnetic field. This magnetic field is perpendicular to the direction of motion of the charge and forms closed loops around the charge's path. The strength of this magnetic field depends on the velocity of the charge, the charge's magnitude, and the distance from the charge. In this article, we will explore the concept of a moving charge and how it produces magnetic fields.
The concept of a moving charge was first discovered by Hans Christian Oersted in 1820. He performed an experiment where a wire carrying a current was placed near a compass. He observed that when the current flowed through the wire, the needle of the compass was deflected. He concluded that a current-carrying wire produces a magnetic field.
This discovery laid the foundation for the understanding of electromagnetism. We now know that a magnetic field is produced by any electric charge in motion. The magnetic field can be visualized using magnetic field lines. These lines indicate the direction of the magnetic field at each point.
If we take a positive point charge and move it along a straight line, it produces a magnetic field around it. The magnetic field lines are directed perpendicular to the direction of motion of the charge. If we move the charge faster, the strength of the magnetic field increases proportionally. We can use the right-hand rule to determine the direction of the magnetic field.
The right-hand rule states that if we point our thumb in the direction of the moving charge, the magnetic field lines circle around the direction of the thumb. If we reverse the direction of the charge, the direction of the magnetic field lines also reverses. If the charge moves in a circular path, the magnetic field lines form concentric circles around the path of the charge.
Now, what happens if we have a group of charges moving in the same direction? The magnetic fields due to each charge add up to produce a stronger magnetic field. We can calculate the net magnetic field by adding the contributions from each charge. The direction of the magnetic field is given by the right-hand rule.
If we have a group of charges moving in different directions, the magnetic fields add up vectorially. The net magnetic field is the vector sum of the individual magnetic fields. This is called the principle of superposition.
One of the most interesting phenomena of a moving charge is the Lorentz force. When a charged particle moves through an external magnetic field, it experiences a force perpendicular to its direction of motion. This force is given by the formula F = q(v x B), where F is the force, q is the charge of the particle, v is its velocity, and B is the magnetic field.
The force on the particle is proportional to the charge of the particle, its velocity, and the strength of the magnetic field. If the particle moves perpendicular to the magnetic field, it experiences a maximum force. If it moves parallel to the magnetic field, it experiences no force.
The Lorentz force is responsible for a wide range of phenomena in nature, from the motion of charged particles in the earth's magnetic field to the function of the cyclotron, a particle accelerator. It is also responsible for the formation of the aurora borealis, or Northern Lights.
In the case of the cyclotron, a charged particle is accelerated in a fixed magnetic field. As the particle moves in a circular path, it is continually accelerating, gaining energy. This energy can be used, for example, to produce medical isotopes.
The Northern Lights are a result of energetic particles from the sun interacting with the earth's magnetic field. When these particles collide with the earth's atmosphere, they produce light. The shape and color of the aurora depend on the energy and type of particle, the altitude of the collision, and the strength and direction of the earth's magnetic field.
Another important aspect of a moving charge is its effect on a current-carrying wire. When a current flows through a wire, it produces its own magnetic field. If a moving charge comes near the wire, its magnetic field interacts with the wire's magnetic field, producing a force on the charge.
This force is given by the formula F = Il x B, where F is the force, I is the current, l is the length of the wire, and B is the magnetic field. The force is perpendicular to the wire and the magnetic field, and its direction is given by the right-hand rule.
This phenomenon is used in many practical applications such as electric motors, generators, and transformers. In electric motors, a rotating magnetic field is produced by a current-carrying wire. The magnetic field interacts with permanent magnets, producing a torque on the rotor, causing it to spin.
In a generator, the opposite occurs. A rotating magnetic field is produced by a mechanical source, such as a turbine. The magnetic field induces a current in a wire, producing an electrical potential. This is used to generate electricity.
Transformers operate on the principle of electromagnetic induction. When a current flows through a wire, it produces a magnetic field. If a second wire is placed nearby, the magnetic field induces a current in the second wire. This principle is used to step up or step down the voltage in electrical systems.
In conclusion, the concept of a moving charge and its effects on magnetic fields are essential to our understanding of electromagnetism. The Lorentz force, the interaction of magnetic fields, and electromagnetic induction are some of the most important phenomena in physics, with many practical applications. Understanding these principles is crucial to advancing technology, from medical imaging to renewable energy sources.