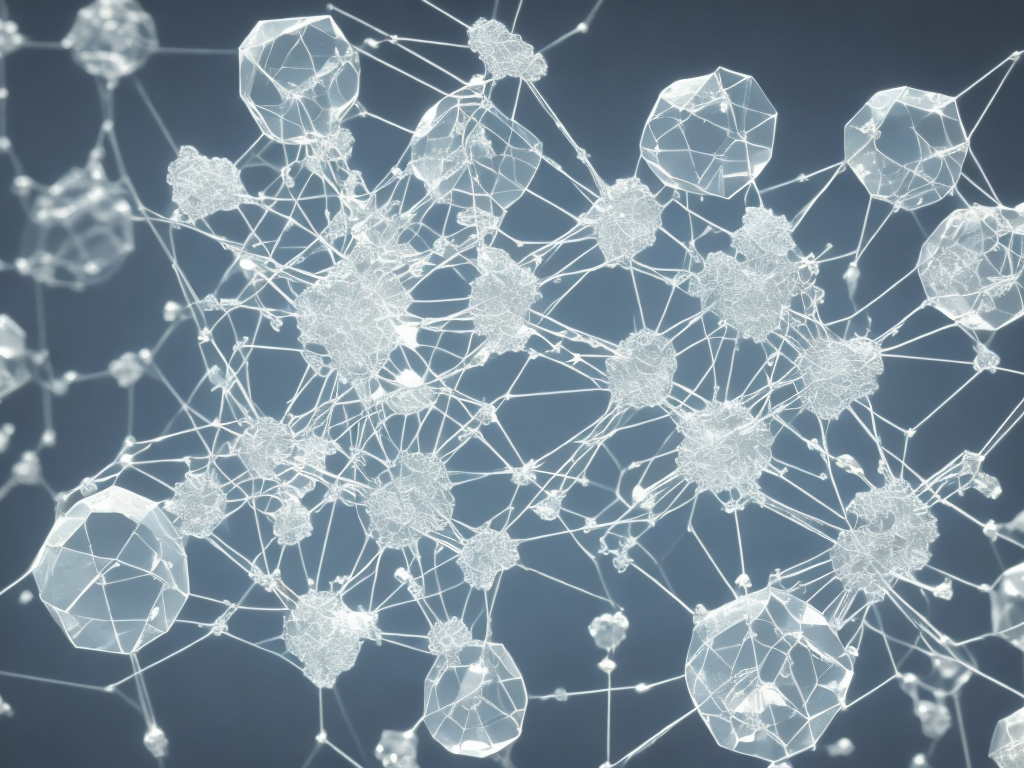
Germanium is an element that has an atomic number of 32 and is a lustrous, brittle, and shiny metalloid. One of the critical properties of germanium is its semiconducting nature, which is essential in electronic devices. This semiconducting property is mainly due to the forbidden energy gap of germanium.
The forbidden energy gap of germanium is the energy gap that exists between the valence and the conduction bands of germanium. The valence band in germanium is the highest-energy band that contains the electrons that are bound to the germanium atom. The conduction band, on the other hand, is the lowest-energy band that contains the electrons that are free to move about the crystal lattice. The electrons in the valence band cannot contribute to electrical conductivity as they are tightly bound to the atoms. However, the electrons in the conduction band can move throughout the entire lattice, contributing to electrical conduction.
The forbidden energy gap is the energy range between the valence and conduction bands where there is no energy level available for electrons. This means that electrons cannot occupy this energy range in the absence of an external energy source. In other words, the electrons in the valence band cannot jump directly to the conduction band without an external energy source that provides the necessary energy to cross the energy gap.
In germanium, the valence and conduction bands are very close in energy, with an energy gap of 0.7 eV. This energy gap is relatively small compared to other semiconductors such as silicon, which has an energy gap of 1.1 eV. Due to this small energy gap, germanium can conduct electricity at a much lower temperature than silicon or other semiconductors.
The forbidden energy gap of germanium has significant implications on its electronic properties. When germanium is doped with impurities such as phosphorus or arsenic, it can become an n-type semiconductor. The dopant atoms introduce additional energy levels within the energy gap, creating a new energy level that is available for electrons to occupy. The impurity atoms contribute an additional electron to the crystal lattice, making it easier for electrons to move to the conduction band. When a voltage is applied to an n-type germanium crystal, the electrons occupy the new energy level introduced by the impurity atoms and contribute to electrical conductivity.
Similarly, when germanium is doped with impurities such as boron, it becomes a p-type semiconductor. Boron atoms have one less electron in their outermost shell, creating a missing energy level or a hole within the energy gap. Electrons from the valence band can jump to the hole, leaving behind a positively charged particle or a hole in the valence band. The hole can represent a positive charge and contribute to electrical conductivity similar to the movement of electrons in an n-type germanium crystal.
In summary, the forbidden energy gap of germanium plays a critical role in its electronic properties. Its small energy gap allows germanium to conduct electricity at a much lower temperature than other semiconductors. It also allows germanium to be doped with impurities to form n-type and p-type semiconductors, making it an essential component in electronic devices such as diodes, transistors, and solar cells. The understanding and manipulation of the forbidden energy gap of germanium have revolutionized the field of electronics and paved the way for the development of modern technology.